Introduction
Campylobacteriosis is a foodborne disease that causes diarrhea with abdominal pain and in some cases, Guillaine-Barre syndrome, which results in pneumatic failure and serious damage to the nervous system [1]. According to the European Food Safety Authority and the European Center for Disease Prevention and Control, campylobacteriosis was ranked as the most common zoonosis in Europe in 2014, with 214,268 confirmed cases [2]. Likewise, the number of reported cases of human campylobacteriosis was 246,307 in 2016, which represents 66.3 cases per 100,000 individuals [3]. The most-reported species causing campylobacteriosis in humans is Campylobacter jejuni and Campylobacter coli, the latter of which has been largely ignored despite the considerable health damage it causes [4]. Contact with live poultry and consumption of raw poultry have been defined as the main routes of Campylobacter infection [4]. Therefore, new rapid detection methods to accurately determine the presence or absence of Campylobacter spp. in chicken or environmental samples are needed [1].
AuNPs were stable, maintaining a red color. When they aggregated, the stable state of AuNPs was interrupted, resulting in a color change to purple or blue. The main point was that AuNPs could be modified by thiolated polymerase chain reaction (PCR) products, which protected AuNPs from salt-induced aggregation [5,6]. Because of this attribute, we modified unique Campylobacter-specific primers including an unlabeled reverse primer and a thiol-labeled forward primer to amplify the target gene [7]. Therefore, thiol-labeled target gene sequence was obtained. After mixing AuNPs with thiolated PCR products, AuNPs were surrounded by a thick layer that consisted of dsDNA. Therefore, this structure tended to change color from red to blue [7]. To obtain a successful result, GNPs need a thick obstacle with plenty of negative charges to prevent their aggregation.
Recently, specific nanoparticles, including silver nanoparticles (AgNPs) and gold nanopartricles (AuNPs), have been widely used as components of new technologies to detect pathogens and hazardous materials using direct or indirect analytes, such as target DNAs, other molecules, or aptamers, which has been carried out in our previous research [8–10]. When various solutions change from dispersion to aggregation state, specific nanoparticles exhibit significant color change due to their unique optical properties, which can be observed using a UV-visible wave-based spectrophotometer or the naked eye [11,12].
Consequently, Campylobacter is well established as a major source of bacterial foodborne pathogens worldwide. The various symptoms of campylobacteriosis can range from mild to severe both in children and the elderly. This pathogen exists as a cytochrome oxidase-positive, hippurate hydrolase-positive, microaerophilic, curved gram-negative rod that exhibits corkscrew motility. It can infect and be transferred from the intestines of a number of wild and domestic animals, particularly avian species such as poultry. Hence, this review aims to elucidate and discuss the pathogenesis of Campylobacter spp. and to highlight the applicability of biofunctionalized nanoparticles for the rapid detection of foodborne pathogenic bacteria.
Campylobacter Virulence Factors
Virulence mechanisms have not been specifically characterized for Campylobacter spp. due to the lack of pathogenic similarity between campylobacters and other pathogens [13]. Flagella-mediated motility, bacterial adsorption in the intestinal mucosa, invasive ability, and the possibility of toxin production have all been reported as potential virulence factors [13,14].
Additionally, limited information regarding this pathogen has confirmed that flagella are required for intestinal colonization [15]. Invasion causes cellular inflammation that subsequently results in the production of cytotoxins, and this followed by a reduction in the absorptive ability of the intestine. Based on this, Campylobacter are then able to reach the intestinal tract. Moreover, due to the resistance of this pathogen to gastric acids and bile salts, disease severity may also be dependent upon the immune condition of the host [16].
Flagella
Motility, a process that increases under highly viscous conditions, is necessary for the colonization of the small intestine [17]. Additionally, the function of flagella under different chemotactic conditions is critical for bacterial survival in various ecological sites that are encountered within the gastrointestinal area [18].
The flagellum of C. coli consists of two homologous flagellins that include FlaA and FlaB. These are encoded by two flagellin genes that are arranged in tandem. The flaA gene is controlled by the promoter σ28, and the flaB gene is regulated by the dependent promoter σ54 [18]. The flaA gene is regarded as fundamental for the invasion of epithelium, as it is established that a mutation in this gene leads to a truncated flagellar filament composed of flaB that exhibits a severe reduction in its motility. Despite this, a mutation in flaB appears to possess no structural significance compared to the structure of normal flagella [17]. The flaA gene is responsible for the adherence and colonization of the gastrointestinal tract and for invasion of host cells, where it subsequently detains the immune response [18]. It is currently speculated that flagella possess another characteristic (the ability to secrete non-flagellar proteins) that may be involved in the observed virulence phenomenon [15]. C. jejuni possesses a polar flagellum composed of O-linked glycosylated flagellin, which is a two-component system composed of the sensor FlgS and the response regulator FlgR [13].
Chicken Related Campylobacter spp.
Chicken meat is a significant source of proteins, and it is rich in essential amino acids, vitamins, and minerals. Fleshy chicken contains more protein than does the same amount of fleshy roasted beef, and the prices of chicken meat are lower than those of beef or pork [19]. Moreover, chicken by-products are frequently consumed due to their low price, mild taste, and short preparation time. However, the consumption of chicken has resulted in numerous recent outbreaks of chronic campylobacteriosis worldwide in both industrialized and developing countries [20]. Most of these cases occur as a result of touching raw poultry, eating raw or uncooked poultry meat, or cross-contamination from raw to cooked foods [20,21]. According to an observational study in England and Wales, C. jejuni was reported to be responsible for more than 12-fold the number of cases of human campylobacteriosis compared to those caused by C. coli [22].
The intestinal tract of chickens can contain a large amount of Campylobacter spp., and during processing, the intestinal tract may rupture or leak and allow the contents can be transferred to the skin [23]. Campylobacter spp. remain in a liquid layer on the skin and become entrapped within the cervix [24]. The tenacity and survival of Campylobacter spp. Are both promoted by the appropriate microenvironment located on the skin of chickens, and even under frozen conditions or storage at 4°C, Campylobacter spp. can remain in the chicken carcasses [24,25].
A study was performed to investigate the effect of temperature during different seasons on the survival of C. jejuni in poultry. Wills and Murray demonstrated that Campylobacter spp. represented a persistent concern in poultry, particularly during warm months (May through October) [26]. During this period, 87%–97% of the tested samples were positive for C. jejuni. The lowest number of positive samples was confirmed in December (7%) and January (33%) [26].
Application of Biofunctionalized Nanoparticles (NPs)
Nanoparticle technologies have been extensively used for effective and rapid detection of various microorganisms; however, there are a few limitations that can be mitigated or avoided completely by understanding the critical parameters involved in these reactions. This review addresses the primary parameters regarding the fusion technology of nanoparticles and the various biosynthesis methods and provides a reasonable categorization of the current approaches with particular focus on gold nanoparticles (AuNPs) and silver nanoparticles (AgNPs) (Figs. 1 and 2).
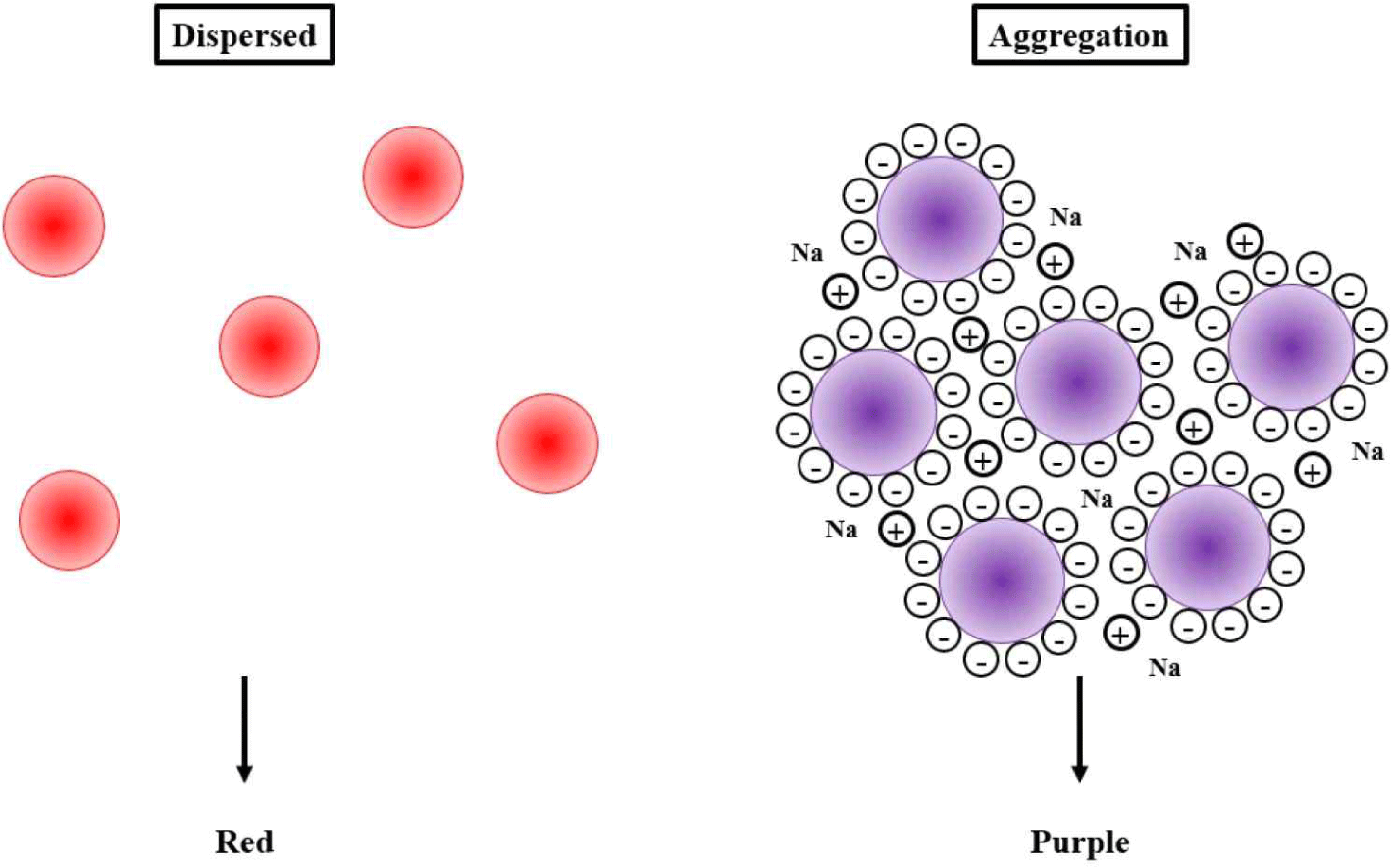
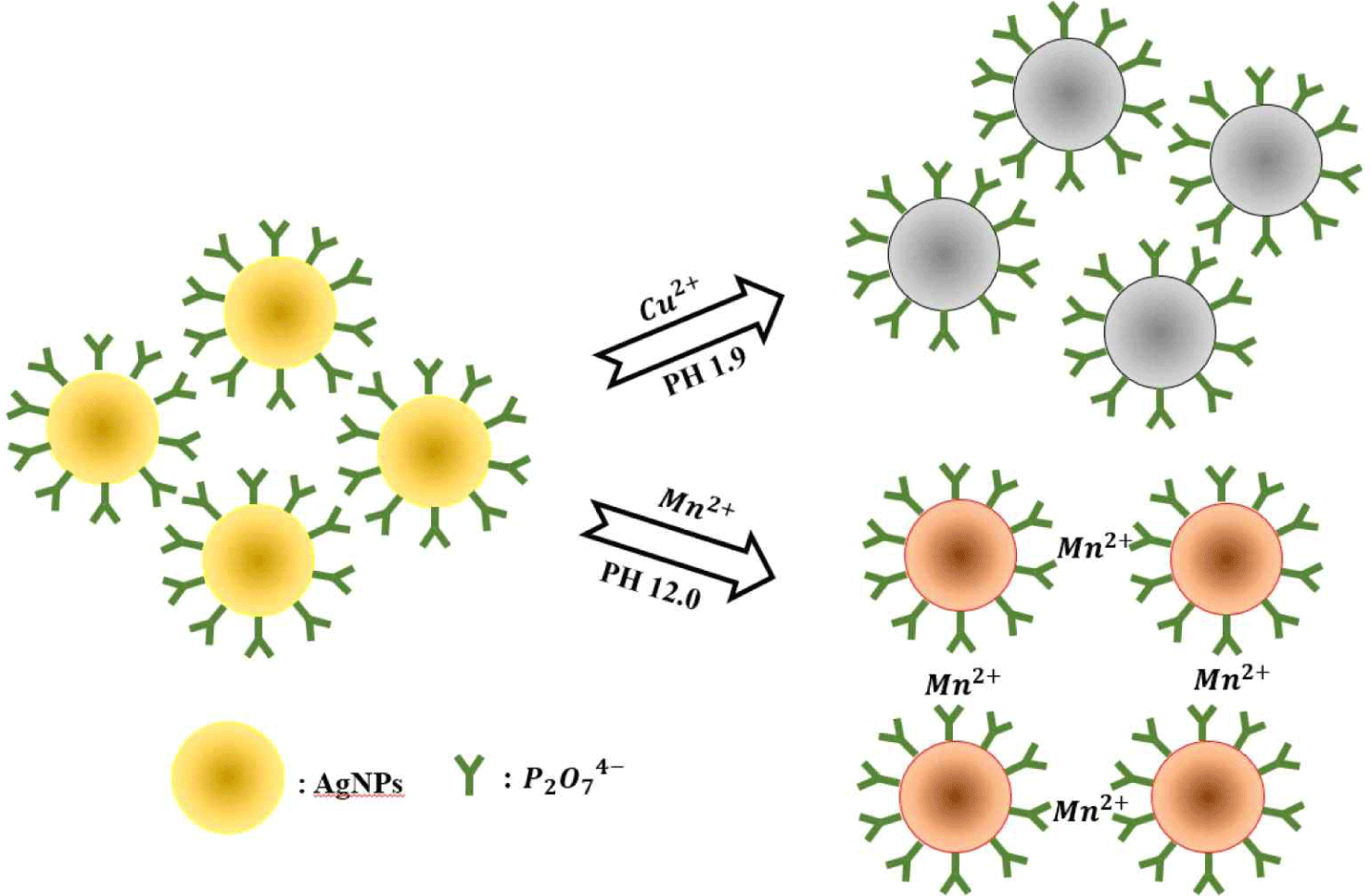
The AgNP- and AuNP-based colorimetric assays can be very efficient and sensitive, particularly for biomolecule identification and the detection of foodborne pathogenic bacteria in environmental samples. In particular, this review highlights the ability to detect pathogens through their coordination with nanoparticle-stabilizing ligands. This review also presents various approaches that are based on label-free aptamers and PCR products to better understand their role as smart sensing devices.
The term “Nanoparticle (NP)” defines any small object ranging in size from 1 to 100 nm that behaves as a unit in regard to its transport and properties. It is established that nanoparticle properties change as a function of particle size, and based on this, these particles can be classified according to their diameter. Thus, ultrafine particles (or NPs) are particles with diameters of between 1 and 100 nm, while fine particles possess diameters of between 100 and 2,500 nm. For tailored applications, NPs can be capped with a variety of anionic and cationic ligands that can range from displaceable small molecules to polymer coatings. The choice of capping ligand depends upon the type of NPs that are used, and these can range from conductive inks to biomedical tools [27].
Among the known nanoparticles, gold and silver NPs (AuNPs and AgNPs) have been widely studied due to their unique optical, electrical, and photothermal properties. AuNPs and AgNPs exhibit unique optical features in well-dispersed solutions, and these features are dependent upon their level of aggregation, a property that is primarily determined by their specific surface plasmon resonance (SPR) profiles [28]. Metal-noble NPs are of a small enough size to confine their electrons and produce quantum effects. This is a key parameter for naked-eye colorimetric sensing applications, as modifications of their surface charge are transformed into a visible color change. Furthermore, NPs also possess very high extinction coefficients that depend primarily upon their size, shape, and inter-particle distance. Such properties enable NPs to compete with analytical techniques such as absorbance or fluorescence spectroscopy. Colorimetric-based assays have been developed based on exploiting the color changes that are associated with the aggregation of metal-noble NPs [29]. Based on their adaptability, high sensitivity, low cost, and versatility, AgNP- and AuNP-based assays have been used to detect metal ions, small molecules, proteins, DNA, and enzymes [27,30–33]. AuNPs are often used as sensing elements to develop sensitive, selective, simple, and label-free colorimetric assays [34]. Consequently, the use of NPs as detection agents could be considered as a type of “litmus test” for target molecules [35]. Analytical methods such as high-performance liquid chromatography, electrophoresis, voltammetry, and fluorescence spectroscopy are the preferred approaches for the detection of various macromolecules; however, sensitive, fast, and high-throughput screening methods are still required [27]. NP-based colorimetric methods are rapid and user-friendly detection approaches that can exploit various chemical mechanisms. For example, these methods can be used for the rapid detection of influenza viruses based on the binding between the influenza virus envelope protein hemagglutinin and sialic acid stabilized AuNPs or for high-throughput screening of endonuclease inhibitors [33,36].
In this review, colorimetric approaches that allow for the naked-eye detection of color changes through ultraviolet-visible (UV-Vis) absorption spectroscopy without fluorescence detection methods are addressed [37]. Accordingly, these methods have been subdivided according to the analytes and NP surface modifications and are not based on the NPs used, as the colorimetric performances of AgNPs or AuNPs are highly similar [38].
Characteristics and Morphology of Gold and Silver Nanoparticles (NPs)
The most commonly used methods for the synthesis of AgNPs and AuNPs involve the reduction of AgCl or AgNO3 and HAuCl4 with sodium citrate and sodium borohydride. According to a previous study, the AuNP mixture was boiled with vigorous stirring in a round-bottom flask fitted with a reflux condenser for approximately 10 min, and a color change from yellow to wine red was observed within seconds [39]. The AuNP solution concentration was calculated following Beer’s law and using the extinction coefficient of 2.7 × 108 M–1 cm–1 at λ 520 nm [40]. For AgNPs, 1% trisodium citrate was added to a 0.3 silver nitrate solution, and the mixture was stirred for 5 min. After the dropwise addition of 1 mM sodium borohydride solution in the dark, the resulting mixture was stirred at room temperature for 2 h. The bright yellow AgNPs were filtered through a Millipore syringe (0.45 nm) to remove the precipitate. NPs were then characterized using diffusion light scattering to calculate their hydrodynamic radius in solution or by transmission electron microscopy to describe their morphology.
For colorimetric assays, AgNPs possess certain advantages over AuNPs. Specifically, AgNP extinction coefficients are higher than are those of AuNPs of the same average size; however, AuNPs are more popular. This could be explained by the observation that AgNP functionalization typically leads to their chemical degradation and, thereafter, the AgNP surface can be easily oxidized, thus reducing their stability [41]. Indeed, Mancuso et al. reported that AuNPs are stable for longer than 1 month at room temperature, while AgNPs are stable for only approximately two weeks [42]. This difference may be linked to the different reaction constants of thiolated gold and silver. The high extinction coefficients and distance-dependent optical properties of AuNPs account for the high sensitivity of AuNP-based colorimetric assays. Moreover, color changes can be easily observed by the naked eye, and this makes these particles attractive for use in DNA-related colorimetric assays [34]. The kinetics of DNA adsorption by AgNPs are slower than are those of AuNPs, and they cannot be accelerated by adding salt at neutral pH. This unique property regarding the specific molecular recognition of DNA-related colorimetric assays accounts for the difficulty of attaching DNA to AgNPs at neutral pH [43].
Similarly, AgNPs are good candidates for use as optical sensors based on their ability to display distance-dependent optical properties [36]. Their stability can be improved by producing Ag/Au core-shell NPs that retain the Ag core optical properties. However, oligonucleotide-modified Ag/Au alloy particles are not as stable as are oligonucleotide-modified core-shell particles, as they irreversibly aggregate under comparable conditions. Furthermore, nanoparticles such as nanorods, prisms, and bipyramids exhibit different SPR wavelengths [42]. Therefore, sensing platforms based on the optical properties of AuNPs in combination with the molecular recognition of ligands such as alkyl thiols, antibodies, nucleic acids, and proteins are active areas of research. Mirkin et al. developed aptamer-based colorimetric assays for macromolecules using more stable AuNPs, despite AgNPs exhibiting a greater extinction coefficient [44].
NP colloidal stability can be adjusted by modifying the surface charges that affect electrostatic stabilization, and NP aggregation can be induced through the loss (or screening) of surface charges. When AgNPs and AuNPs are exposed to light, they oscillate in the electromagnetic field of light. This induces a collective coherent oscillation of the conduction band electrons, thus resulting in SPR. The SPR band intensity and wavelength are dependent upon factors that affect the electron charge density on the particle surface. According to Mie’s theory, these factors include the metal type, the particle size, shape, structure, and composition, and the dielectric constant of the surrounding medium [45].
Thus, unmodified AuNPs are red, while AgNPs are blue or maroon due to their specific and size-dependent SPR absorption. The addition of salt to the reaction triggers electrostatic repulsion between negatively charged NPs and antiparticle changes, ultimately resulting in NP aggregation and subsequent specific color and wavelength changes [46]. Hence, by monitoring the changes in absorbance, it is possible to understand the characteristics of the enhanced scattering effect in aggregated NPs compared to that of non-aggregated NPs [47]. Consequently, the band gap energies can also be used to improve the understanding of NP sensing and catalytic properties [48]. The SPR profile is characteristic of NP surface modifications induced by small molecules, metal ions, and bio-macromolecules [49].
Conclusion
The conventional method for detecting Campylobacter spp. requires a lot of experimental time, cost, and labor, and additional analysis through PCR is essential. Above all, a simple and rapid detection method for accurately determining the presence of Campylobacter in environmental samples or chickens is urgently needed. Up to now, the method for detecting Campylobacter spp. in chicken carcasses and chicken feces using AuNPs in a colorimetric biosensor has been known. First, PCR products and colloidal AuNPs are mixed, and then the thiolated PCR products bind to the AuNP surface to form gold nanoparticle-PCR (GNP-PCR) products. This reaction is because the thiolated PCR products have abundant negative charges, so they not only attach well to the AuNPs, but also maintain a dispersed form under electrostatic repulsion. This method provides color differentiation of AuNPs, and has the advantage of not requiring additional time or pH optimization steps for the thiolated PCR products to attach to the AuNPs. Therefore, it is evaluated that this platform will provide an efficient detection method for various food poisoning bacteria.