Introduction
Yogurt is popular dairy product obtained by fermentation of lactose to lactic acid by lactic acid bacteria (LAB), and can be made from all types of milk [1]. The food industry commercial probiotics are Lactobacillus and bifidobacteria used in products such as yogurt, milk powder, cheese, frozen desserts and dairy [2]. These are living microorganisms that have a positive effect on the health of the host when consumed in appropriate amounts [3,4]. Probiotics lower gut pH, release gut protective metabolites, and achieve appropriate levels of viable cells (>106) are the subject of research, and food industries are exploring ways to add new probiotic strains to foods and introduce new technologies [5,6].
Nobel laureate Eli Metchnikoff (1845–1916) said that “the majority of diseases begin in the digestive tract when “good” bacteria are no more able to control “bad” bacteria”, calling this condition dysbiosis [7]. Modern lifestyle, bad eating habits, stress, lack of exercise and improper use of antibiotics cause dysbiosis of the gastrointestinal tract, which is often the beginning of non-infectious chronic diseases. The above non-communicable diseases have increased the demand for probiotic functional food market, the popularity of probiotics continues to grow, and the production of various food industry continues to grow rapidly [3,8,9].
Milk and dairy products, especially yogurt, effectively introduce probiotics and prebiotics into the human’s digestive system. It is also resistant to gastrointestinal hostility and has antioxidant and antagonistic activity, making it a sought-after product worldwide [2,9,10–12]. Yogurt culture improves lactose digestion and relieves symptoms of intolerance, so there’s an exciting new option for vegetarians, lactose intolerants, and those with milk allergies plant-based yogurt [1,13]. Plant-based yogurt refers to a product fermented with a starter LAB isolated from plants. Therefore, nutritional recommendations aimed at maintaining physical and mental health help to absorb plant LAB and proteins more intensively [14,15].
Among the positive effects of LAB used in the fermentation of dairy products, we can mention a decrease in pH, an increase in antioxidant activity, the production of antimicrobial metabolites and exopolysaccharides, the formation of flavors and aromas, the detoxification of mycotoxins, and the formation of vitamins and bioactive peptides [16]. We have previously identified and identified blueberry LAB and confirmed its effectiveness against pathogenic bacteria [15]. Streptococcus thermophilus and Lactobacillus delbrueckii subsp. bulgaricus are the main LAB that affect the final taste and nutritional quality of yogurt [17–19]. Therefore, considering the limited biological diversity of commercial starter culture, the aim is to obtain a new culture to study the synergistic effect of the above LAB with L. argentoratensis strain isolated from blueberries.
Materials and Methods
S. thermophilus KCCM 40430 and L. delbrueckii subsp. bulgaricus KCCM 35463 were obtained from the Korean Culture Center of Microorganisms and used in this study. The commercial strain YC-380 (S. thermophilus, L. bulgaricus) was purchased from Chr. Hansen (Denmark) and utilized. The probiotic strains were cultured for two generations to enhance their activity before being used in the experiments. The culturing medium for these strains was De Man Rogosa-Sharpe broth (Difco, USA).
Single strain fermented milk was prepared by inoculating each strain into pre-sterilized triangular flasks containing 400 mL of commercial milk and incubating at 37°C for 48 hr. S. thermophilus, L.bulgaricus, and L. argentoratensis (isolated from blueberry) were inoculated at a concentration of 2% each. Mixed strain fermented milk was prepared by inoculating each strain into pre-sterilized triangular flasks containing 400 mL of commercial milk and incubating at 37°C for 48 hr. L. argentoratensis and YC-380 were mixed inoculated at a concentration of 2% each, and as a control, YC-380 was inoculated at total 2% concentration and fermented at 37°C. The milk was incubated at 37°C until reaching a pH of 4.5, with measurements taken every 3 hr.
During fermentation, pH and titratable acidity were measured at 3 hr intervals over a period of 48 hr. pH was measured using a pH meter (Mettler-Toledo, USA), while titratable acidity was determined according to the Association of Official Agricultural Chemists (AOAC) method. 17.6 mL of fermented milk was diluted 1:1 with distilled water, and 0.1N NaOH was added until the pH reached 8.3, at which point the acidity was measured.
During fermentation, the enumeration of viable cell counts was performed at 12 hr intervals. After evenly mixing the fermented milk, 1 mL of each sample was diluted with 9 mL of sterile 0.1% (wt/vol) peptone water and serially diluted. Then, 1 mL of each diluted solution was plated onto MRS agar (Difco). After incubating at 37°C for 24 hr, the number of colonies formed was counted, and the colony-forming units (CFU) per milliliter of sample were calculated as the average of triplicate.
Viscosity measurement was conducted after the completion of fermentation. 40 mL of the sample was taken, and viscosity was measured using NDJ-8S Digital Rotational Viscometer (W&J Instrument, China) with Spindle No. 4. After achieving sufficient stability, measurements were taken three times at 30 rpm with 1 min intervals, and the average value was recorded.
For the measurement of DPPH free radical scavenging activity, the fermented milk and the control were mixed with ethanol in a 1:1 ratio. The resulting supernatant obtained by centrifugation (Hanil Centrifuge, Korea) at 4,000 rpm for 20 min was used as the sample solution. To assess the DPPH radical scavenging ability, 0.2 mM 2,2-diphenyl-1 carboxylic acid dissolved in ethanol was mixed with the cell-free supernatant in a 1:1 ratio and allowed to reached for 30 min in the dark room. Further, absorbance was measured at 517 nm using the Epoch Microplate Spectrophotometer (Biotech, USA). The mixture of supernatant and methanol was used as a blank. The percentage inhibition (%) of DPPH radical scavenging activity was calculated according to the following formula:
The acid resistance of L. argentoratensis isolated from blueberries was evaluated. After culturing L. argentoratensis for 12 hr, the pH was adjusted to 2.0 using 1 N HCl. The viable cell count was measured after 60 and 120 min of exposure to pH 2.0.
After fermentation, the single strain (S. thermophilus, L. bulgaricus, and L. argentoratensis) inoculated fermented milk is stored at 10°C. Changes in pH, total titratable acidity, and viable cell count were measured at 6 day intervals over a period of one month.
Results and Discussion
Fig. 1 shows the changes in pH and titratable acidity during the 48 hr incubation of milk inoculated with each strain at 37°C. The S. thermophilus strain reached a pH of 4.5 the fastest at 30 hr of incubation. However, L. bulgaricus and L. argentoratensis reached a pH of 4.5 at 48 hr of incubation (Fig. 1A). Meanwhile, titratable acidity showed the same trend as pH, with S. thermophilus producing the most lactic acid (Fig. 1A). L. bulgaricus and L. argentoratensis followed in that order (Fig. 1A). Fig. 1B shows the viable cell count of each strain at 12 hr intervals during the 48 hr incubation. After 12 hr of incubation, S. thermophilus, L. argentoratensis, and L. bulgaricus showed 9.13 Log CFU/mL, 8.98 Log CFU/mL, and 8.69 Log CFU/mL, respectively (Fig. 1B). At 48 hr of incubation, the viable cell counts of S. thermophilus, L. argentoratensis, and L. bulgaricus were 9.47 Log CFU/mL, 9.49 Log CFU/mL, and 9.78 Log CFU/mL, respectively. Especially, L. argentoratensis showed a higher pH and lower titratable acidity compared to the S. thermophilus, but had a higher viable cell count than S. thermophilus and L. bulgaricus at 48 hr measured time point (Fig. 1B).
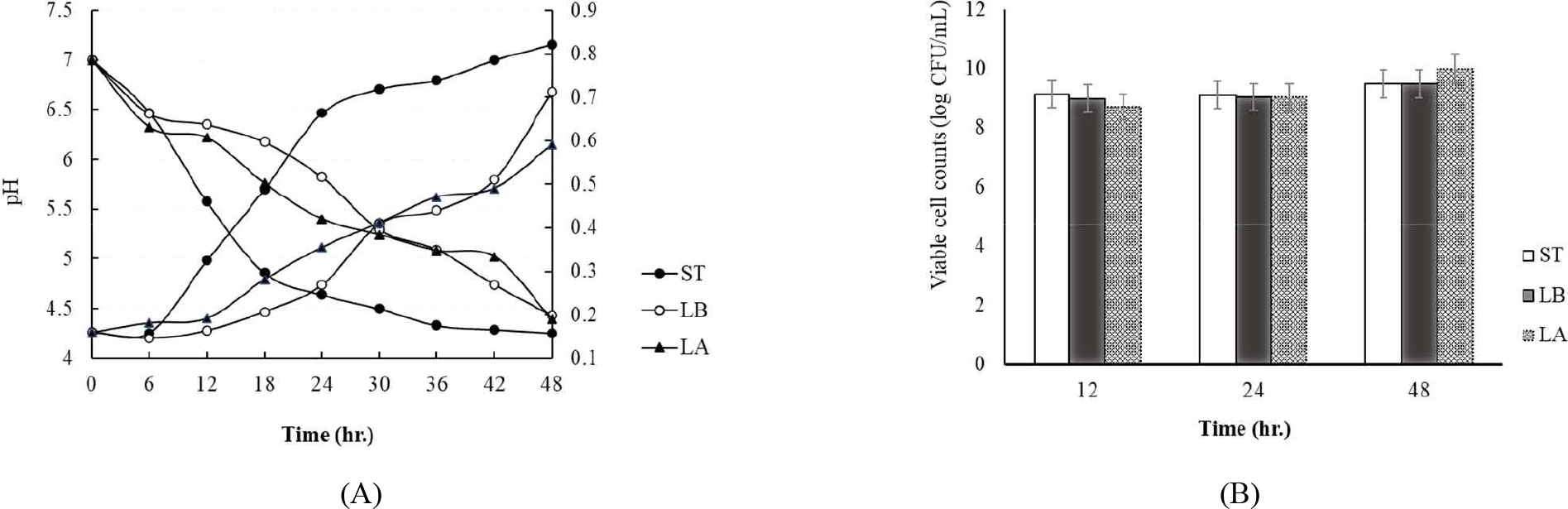
Fig. 2 shows the changes in pH, titratable acidity, and viable cell count during the 24 hr incubation of the commercial strain YC-380 and a mixture of YC-380 and L. argentoratensis. The pH and titratable acidity decreased more rapidly in the test group cultured with the mixture of YC-380 and L. argentoratensis, showing an increase in lactic acid production (Fig. 2A). Fig. 2B shows the viable cell counts of YC-380 alone and the mixture of YC-380 and L. argentoratensis at 12 hr intervals during the 24 hr incubation. At 12 hr of incubation, the viable cell counts were 8.92 Log CFU/mL and 9.63 Log CFU/mL for YC-380 and the mixture, respectively, with YC-380 and L. argentoratensis showing a 1 Log higher count (Fig. 2B). At 24 hours of incubation, the viable cell counts of YC-380 and the mixture were 10.03 Log CFU/mL and 10.36 Log CFU/mL, respectively, with no significant difference between the two groups (Fig. 2B).
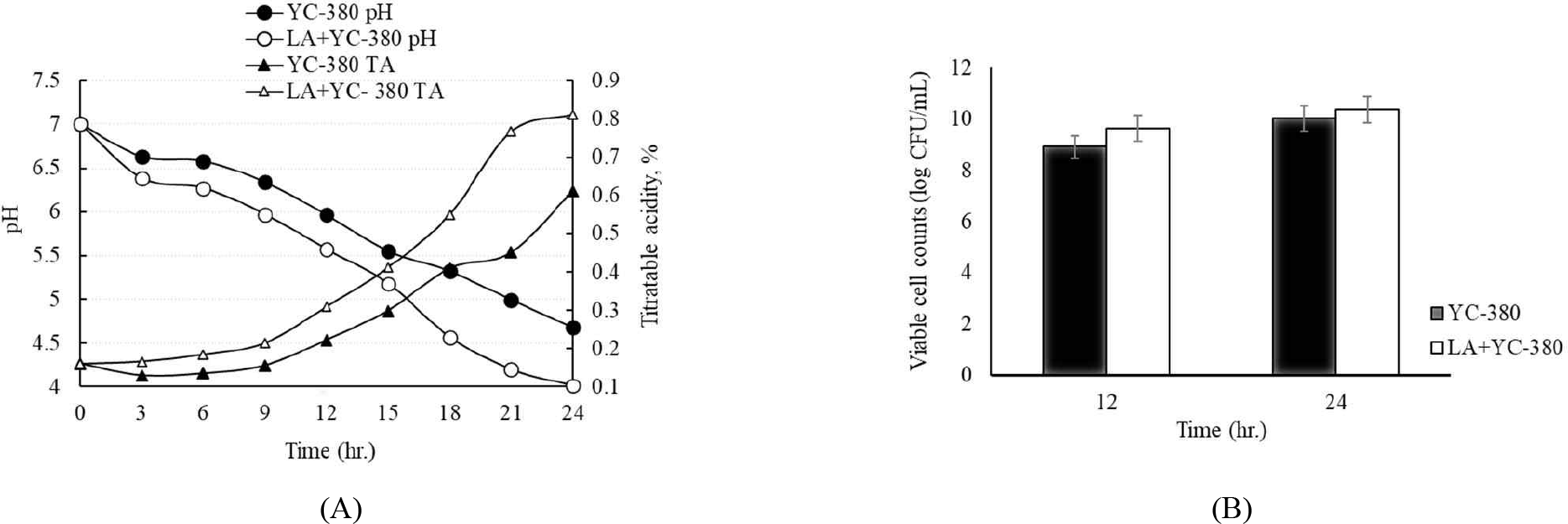
The change in pH has been reported to be due to the varying degrees of hydrogen ion concentration increase, which is attributed to the production of organic acids by LAB using lactose and the increase in peptides, amino acids, and free fatty acids resulting from enzymatic hydrolysis [20]. Due to these characteristics, yogurt is produced through the lactic acid fermentation of milk, primarily using cultures of L. bulgaricus and S. thermophilus [21]. In this study, S. thermophilus and L. bulgaricus exhibited a rapid decrease in pH, whereas L. argentoratensis showed a slower pH change. This is likely due to differences in the utilization of sugars and the production of enzymes involved in hydrolysis by L. argentoratensis.
Figs. 3 and 4 show the antioxidant activity after manufacturing fermented milk from each strain. The antioxidant activity of unfermented milk was 15.2% (Figs. 3 and 4). The antioxidant activity of fermented milk made with single strains was 70.5% for L. argentoratensis, 63.9% for S. thermophilus, and 70.6% for L. bulgaricus (Fig. 3). Thus, fermented milk made with L. argentoratensis showed the highest antioxidant activity. The antioxidant results of the culture fluid of YC-380 and the mixture of YC-380 and L. argentoratensis are shown in Fig. 4. The antioxidant effect of YC-380 was 75.06%, and the antioxidant effect of the mixture of YC-380 and L. argentoratensis was 86.94% (Fig. 4). Therefore, the antioxidant activity increased when yc-380 and L. argentoratensis were co-cultured.
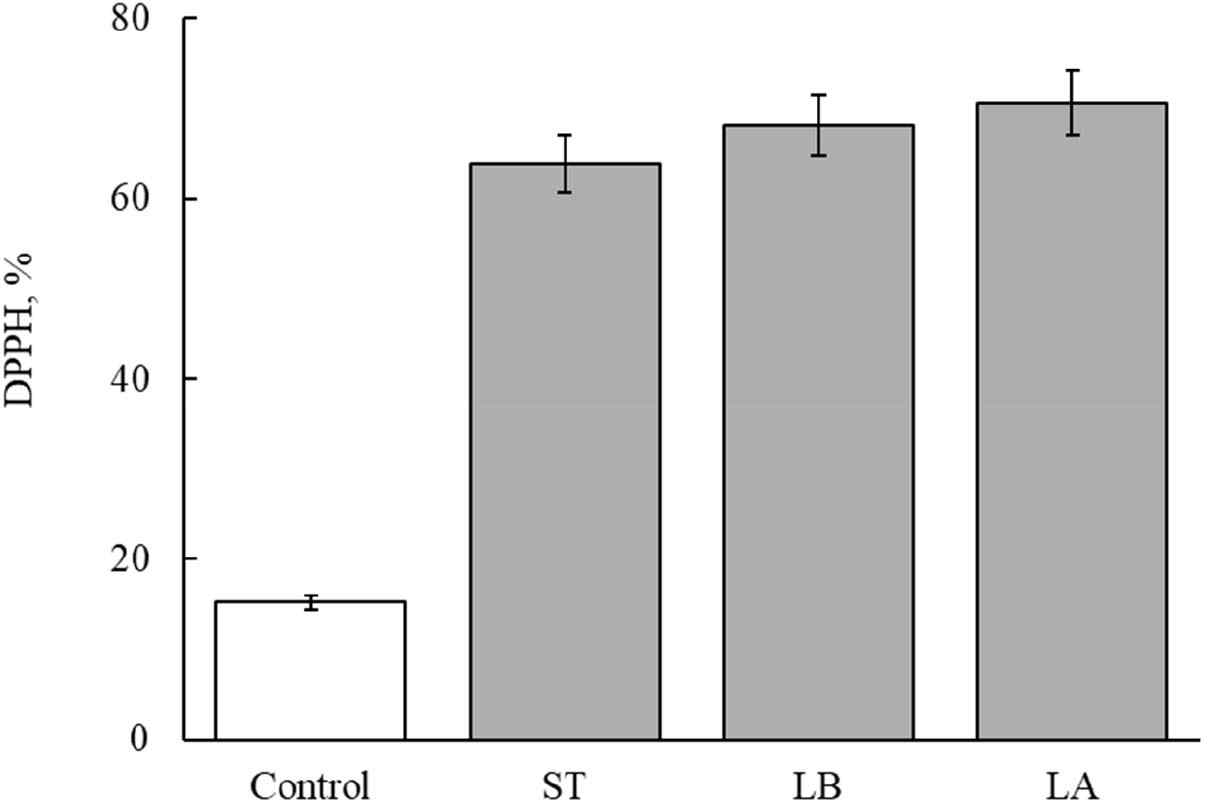
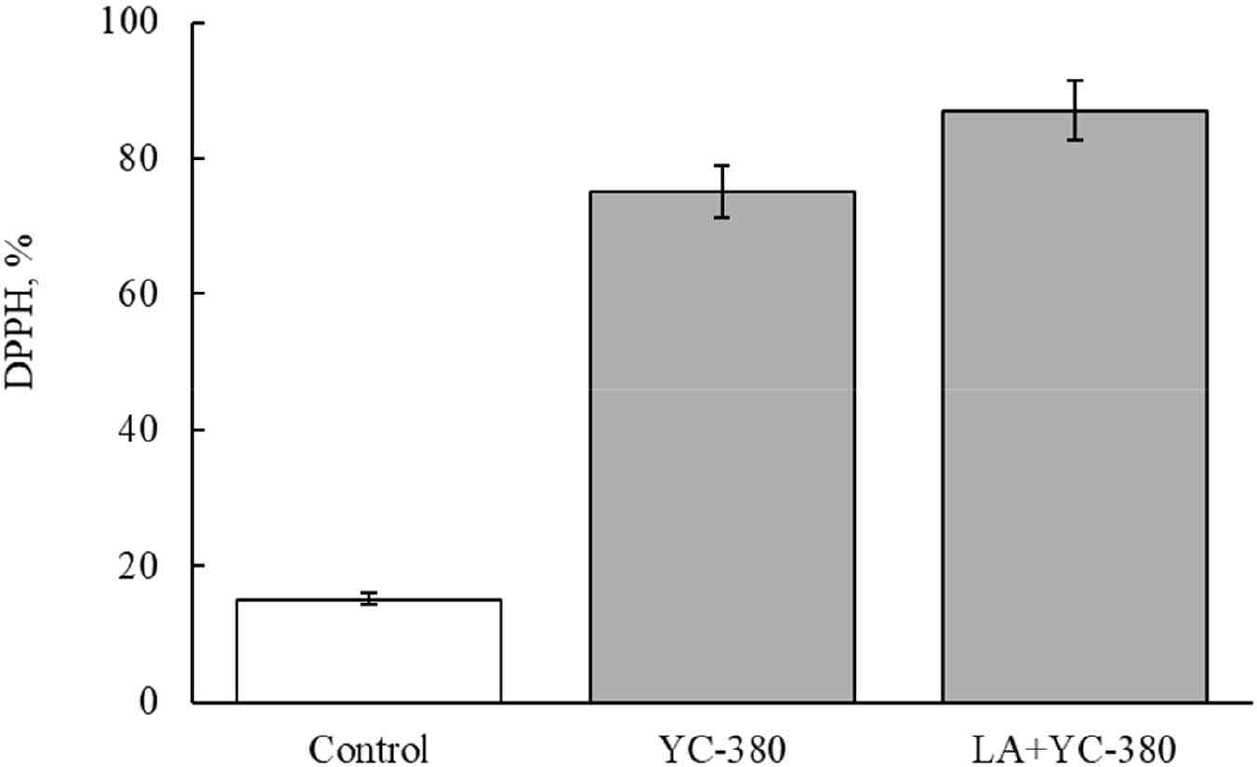
DPPH is a chemically induced, relatively stable radical that is used to measure antioxidant effects by utilizing the fact that the radical is scavenged through a reduction process when it receives electrons from antioxidant substances such as ascorbic acid, tocopherol, polyhydroxy aromatic compounds, and aromatic amines [22]. Proteins found in yogurt whey, such as β-lactoglobulin, α-lactalbumin, bovine serum albumin, lactoferrin, and immunoglobulin, contribute to antioxidant activity [23]. In this experiment, the antioxidant effects of each LAB varied in the milk medium. Therefore, it is believed that, in addition to whey proteins, the metabolic products of the LAB also secrete antioxidant components. Ali et al. [24] reported that the antioxidant ability to scavenge DPPH radicals varies according to the antioxidant enzymes, proteins, and cell surface components produced by Limosilactobacillusreuteri cells.
The curd of fermented milk from each strain was crushed at 30 rpm for 1 min after 24 hr of incubation and again after 48 hr of incubation. The samples were then stored at 5°C for 24 hr. The viscosity measurement results are shown in Fig. 5. At 24 hr, the viscosity was 620 CP for S. thermophilus, 1,030 CP for L. bulgaricus, 640 CP for L. argentoratensis, 764 CP for YC-380, and 997 CP for the mixed fermentation of YC-380 and L. argentoratensis. At 48 hr, the viscosity was 915 CP for S. thermophilus, 1,280 CP for L. bulgaricus, 1,100 CP for L. argentoratensis, 932 CP for YC-380, and 1,480 CP for the mixed fermentation of YC-380 and L. argentoratensis.
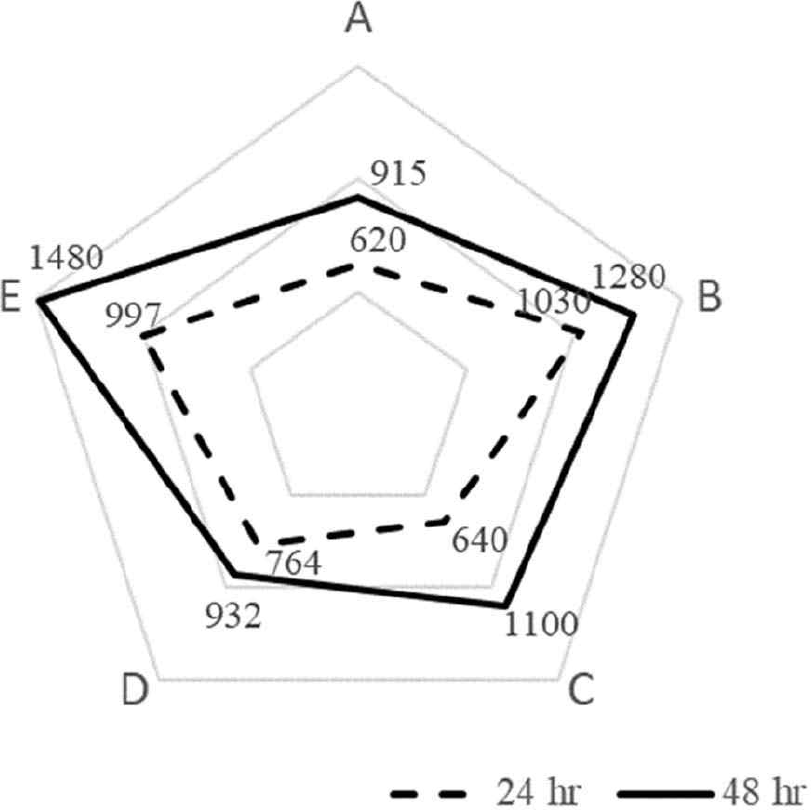
Generally, curd formation, which causes viscosity, is reported to occur through a complex process during lactic acid fermentation, involving isoelectric precipitation of milk proteins, breakdown by proteases, and production of polysaccharides by LAB [25]. In the study by Sung et al. [26], it was observed that as the pH decreases, viscosity also decreases. However, in this study, L. argentoratensis exhibited high viscosity activity despite having a relatively high pH. Rasic & Kurmann [27] reported that the viscosity of fermented milk is influenced by factors such as the total solids, protein content, salt content, acidity, homogenization, and the proteolytic activity of the strains used. In this study, different viscosities were observed depending on the strains used. When yogurt was made using L. argentoratensis in combination with other strains, the curd formation was well-established, and the viscosity was well-maintained, suggesting the potential for producing high-quality yogurt.
The pH of the culture fluid of L. argentoratensis after 24 hr of incubation in MRS broth was pH 3.92. The results of the acid resistance test for L. argentoratensis are shown in Fig. 6. To measure survival under acidic conditions, the culture fluid was adjusted to pH 2.0 with 1N HCl and exposed for 1 or 2 hr. The viable cell count of the culture fluid exposed to each time period is shown in Fig. 6. The viable cell count immediately after incubation was 7.65 Log CFU/mL, and after 1 hour of exposure to pH 2.0, it was 7.17 Log CFU/mL. After 2 hr of exposure to pH 2.0, the viable cell count was 4.49 Log CFU/mL. Therefore, L. argentoratensis showed high survival under acidic conditions.
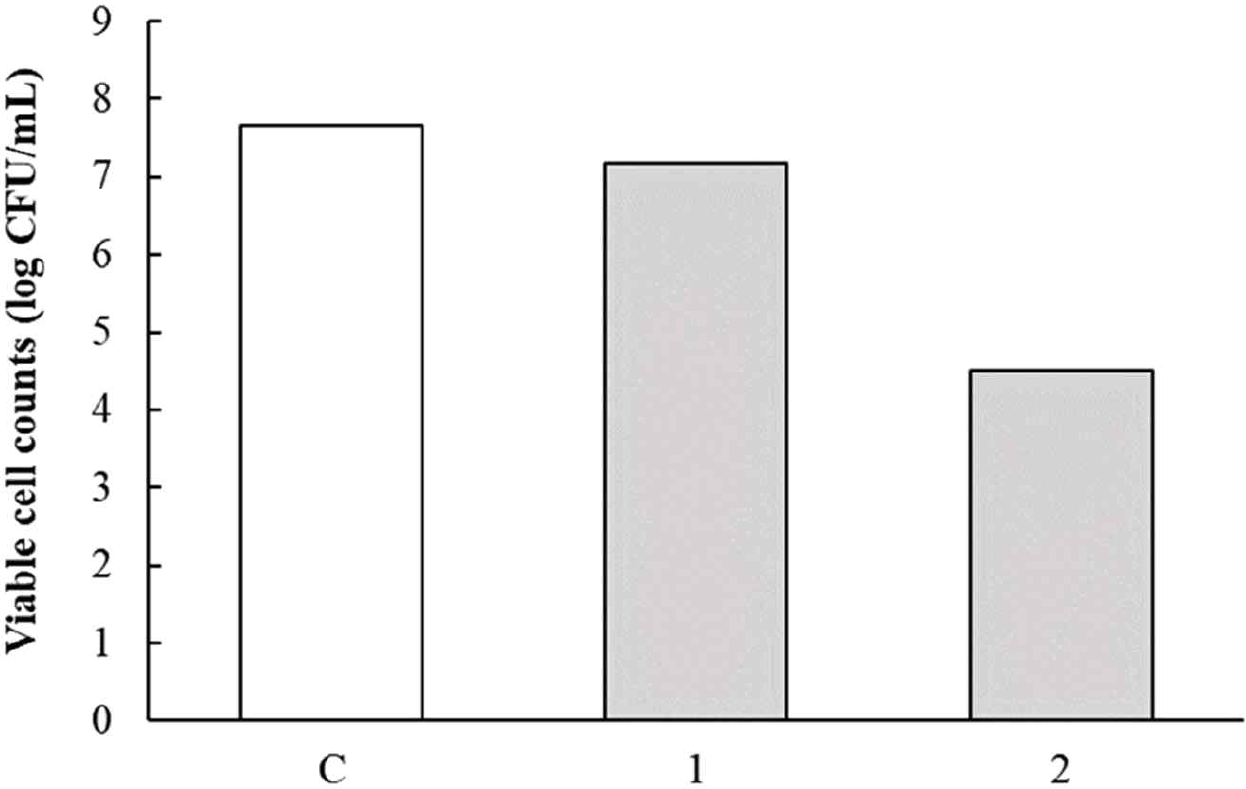
For LAB to be effective as probiotics with benefits such as intestinal regulation, they must be able to survive in acidic conditions and in the bile-rich environment of the digestive tract [28,29]. According to Martini et al. [30], considering that the pH of the stomach rises above 3.0 after the consumption of food or dairy products, the survival rate of these bacteria is expected to be higher. Conway et al. [31] reported that the animal-derived lactic acid bacterium L. bulgaricus cannot survive for more than two hours at pH 3. This result aligns with our findings, where L. bulgaricus showed no viability after being exposed to pH 2 for two hours (data not shown). Additionally, Jeong et al. [32] noted that plant-derived LAB exhibit strong acid resistance, which was corroborated by the high survival rate of L. argentoratensis in our experiment.
Yogurt was made after inoculating S. thermophilus, L. bulgaricus, and L. argentoratensis. The yogurt was stored at 10°C for 30 days, and the pH, titratable acidity, and viable cell count were measured. Fig. 7A and B show the changes in pH and titratable acidity over 30 days of storage. S. thermophilus and L. argentoratensis showed an increase in pH and a decrease in titratable acidity up until day 6 of storage, but no significant changes were observed thereafter (Fig. 7A and B). In contrast, L. bulgaricus exhibited a slight decrease in pH until day 12 of storage, with no further changes observed afterward (Fig. 7A). However, in terms of titratable acidity, L. bulgaricus showed a decrease until day 6 of storage, with no changes observed afterward (Fig. 7B). Meanwhile, the viable cell counts showed no significant changes in all tested samples up until day 24 of storage, but a 1 Log reduction was observed by day 30 (Fig. 7C). Vázquez-Román et al. [33] reported that calcium or phosphate compounds might dissolve during refrigerated storage, leading to an increase in the pH of dairy products. Schiffer et al. [34] suggested that the solubility of calcium increases at low temperatures, which could result in calcium reacting with lactic acid to form calcium lactate, thereby increasing the pH. However, in this study, the LAB used were inoculated into the same milk medium, and after yogurt production, the pH was measured during cold storage. Therefore, it is believed that the pH stability was more influenced by the metabolic products of the LAB than by the effects of calcium and phosphate compounds in the medium.
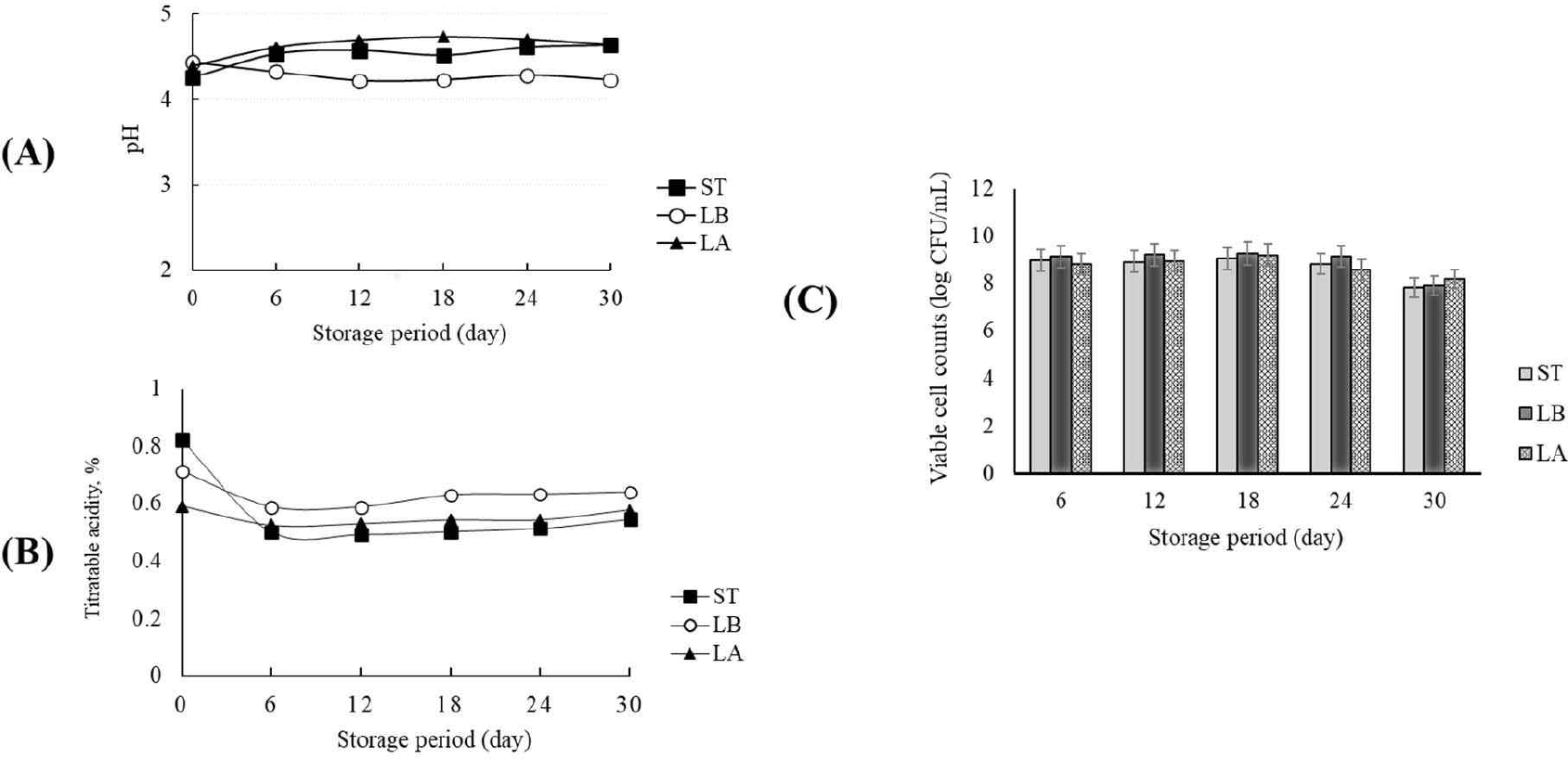
Conclusion
Yogurt is a fermented dairy product made with a starter culture containing S. thermophilus and L. bulgaricus, and is widely accepted as a health food by consumers. Therefore, this study investigated the applicability and probiotic potential of L. argentoratensis, which was isolated and identified from blueberries. L. argentoratensis showed slower pH decrease and lower lactic acid production compared to S. thermophilus when used alone in fermented dairy production. However, its viable cell count was very high despite the slow pH decrease and low lactic acid. Moreover, mixed fermentation of L. argentoratensis with other strains demonstrated rapid pH decrease and high lactic acid production. In terms of viscosity, fermented dairy products containing L. argentoratensis tended to show higher viscosity. After fermentation, the antioxidant activity of the yogurt was highest with L. argentoratensis. The acid resistance of L. argentoratensis was very high, confirming its potential as a probiotic. During 30 days of storage at 10°C, yogurt containing L. argentoratensis maintained stable pH and titratable acidity, with high survival rates. Thus, L. argentoratensis can be used as a new functional starter culture for producing yogurt with health-promoting LAB characteristics.