Introduction
Lactic acid bacteria (LAB) are generally recognized as safe, and many provide nutritional benefits and possess fermentation abilities, which can improve foods’ nutrition, safety, and organoleptic quality [1,2]. In addition, LAB produce organic acids, such as lactic acid, diacetyl, hydrogen peroxide, antimicrobial peptides, and bacteriocins [3], some of which have antimicrobial properties. For example, the lactic acid produced by LAB can inhibit the growth of pathogens and harmful bacteria by decreasing the pH.
Lactococcus is a LAB genus widely used to produce fermented food products [4]. Lactococcus are homo-fermentative, L-lactate-producing bacteria [5], and most species cannot grow above 40°C [6]. Lactococcus lactis is commonly used to produce fermented dairy products [6]. This species produces organic substances that impart aroma and organoleptic properties to fermented foods [7]. Strains of L. lactis are used to manufacture cheese, fermented milk products, and butter [8]. L. lactis has three subspecies: L. lactis subsp. cremoris, L. lactis subsp. lactis, and L. lactis subsp. hordniae. Most strains of the former two subspecies are associated with dairy fermentation. In contrast, L. lactis subsp. hordniae cannot utilize lactose [4].
Furthermore, L. lactis species produce bacteriocins, for example, nisin. Nisin is generally recognized as safe by the United States Food and Drug Administration [9] and is widely used as a preservative in cheese, canned foods, and fermented milk products. However, artificial preservatives are still widely used. Although artificial preservatives are considered safe, several have harmful and potentially life-threatening side effects. For example, artificial preservatives, such as benzoates and nitrates, can cause hypersensitivity, allergy, asthma, and cancer [10].
Bacteriocins are antimicrobial peptides biosynthesized by bacterial genes, unlike, for example, antibiotics, which are secondary metabolites. Bacteriocins typically inhibit microbiologically and morphologically similar bacterial strains, mostly Gram-positive [11]. However, some bacteriocins inhibit Gram-negative bacteria. Bacteriocins are non-toxic, colorless, odorless, and have no residues. Therefore, they are harmless to the human body and can substitute chemical antibiotics in food [12,13].
Bacteriocin classification is currently under dispute. The most common method to classify bacteriocins involves dividing them into three groups. Class I generally comprises 19–50 amino acids that are post-translationally modified, resulting in the formation of the non-standard amino acid lanthionine. Class I has sub-classes Ia, Ib, and Ic, which are lantibiotics, labyrinthopeptins, and sanctibiotics, respectively. The most prevalent Class I bacteriocin is nisin. The mechanism of action of Class I bacteriocins is membrane permeabilization by pore formation. Class I bacteriocins are heat stable. In contrast, Class II bacteriocins are non-modified peptides with low heat stability, which are divided into sub-classes IIa, IIb, IIc, and IId: Class IIa comprises pediocin-like bacteriocins that inhibit Listeria spp.; Class IIb bacteriocins require two different peptides for activation and are unmodified; Class IIc bacteriocins have a circular form; and Class IId are linear and non-pediocin-like bacteriocins. The Class III bacteriocins are large heat-sensitive peptides [14].
As previously mentioned, bacteriocins are mainly effective against Gram-positive bacteria because the outer membrane of Gram-negative bacteria obstructs the bacteriocin action site [15]. Different modes of action have been presented for bacteriocins, including changes in enzyme activity, inactivating anionic carriers, and hindering spore germination [16].
Many Korean studies have investigated bacteriocins that exhibit antibacterial activity. This study aimed to examine LAB and bacteriocins as alternatives to artificial preservatives. Therefore, the antibacterial ability of L. lactis subsp. hordniae JNU533 (JNU533) isolated from Swiss-type cheese, and the characteristics of its bacteriocin were determined. This study’s results reveal that JNU533, similar to other subspecies of L. lactis, could be used as a starter culture for milk products.
Materials and Methods
The JNU533 were isolated from milk products by culturing in deMan, Rogosa, and Sharpe (MRS) broth at 37°C. The isolated-bacterial strain was identified using Gram-staining, morphology, and the potassium hydroxide (KOH) test. Genomic deoxyribonucleic acid (DNA) was extracted using a PureHelix™ Genomic DNA Prep Kit (NanoHelix, Korea). Polymerase chain reaction (PCR) was performed using the universal primers:
27F 5′-AGA GTT TGA TCC TGG CTC A-3′
1492R 5′-GGT TAC CTT GTT ACG ACT T-3′
The cycling protocol was as follows: initial denaturation at 94°C for 5 min; 30 cycles of denaturation at 94°C for 30 s, annealing at 55°C for 1 min, and elongation at 72°C for 1 min. The final cycle was followed by the extention at 72°C for 10 min. The 16S ribosomal ribonucleic acid (rRNA) sequencing was performed by SolGent (Korea) using the Basic Local Alignment Search Tool (BLAST®) for sequence identification.
Additional PCR was performed to clarify that the bacteriocin produced by JNU533 was not nisin using the following primers:
NISR-F 5′-GCATTGGTACTTGCTTTCGG-3′
NISR-R 5′-AACCTTGAGGCGAACGACTT-3′
To prepare a cell-free bacteriocin sample, JNU533 was incubated (1%, 37°C for 24 h), and then the culture was centrifuged at 6,000 ×g for 15 min. The supernatant was filtered (0.2 μm syringe filter; Sartorius AG, Germany) and heated at 65°C for 30 min. The production of bacteriocin was confirmed using Lactobacillus delbrueckii subsp. lactis ATCC4797. Bacteriocin activity was tested using the spot-on-lawn assay with the following indicator strains: Levilactobacillus brevis BMB5, BMB6, OB1, OB3, and OB4; Lactobacillus acidophilus GP1B; Lactiplantibacillus plantarum L67, OY1, and OY2; Limosilactobacillus fermentum; Streptococcus thermophilus; L. delbrueckii subsp. bulgaricus; Listeria monocytogenes; Staphylococcus aureus; Escherichia coli; and Salmonella Typhimurium. Activity units and bacteriocin activity were determined with the highest two-fold dilution showing a clear inhibitory zone on the MRS hard-soft agar plate.
Tricine-SDS-PAGE was performed to estimate the molecular weight of the JNU533 bacteriocin crude extract [17]. First, 100 mL of cell-free bacteriocin sample was saturated by adding ammonium sulfate powder. The samples were stirred at 4 for 5 h to promote precipitation, then centrifuged at 8,000 ×g at 4°C for 30 min. The pellets were resuspended in 5 mL of 4-morpholineethanesulfonic acid (MES) buffer (0.05 M, pH 6.1; Sigma-Aldrich, USA; Merck KGaA, Germany).
The bacteriocin crude extract sample was examined on a 4%–16% tricine-SDS-PAGE gradient gel. The gel was stained with Coomassie Brilliant Blue R-250 (Bio-Rad Laboratories, USA) and washed with destaining buffer (Coomassie Brilliant Blue R-250 Destaining Solution, Bio-Rad Laboratories). In addition, the gel was placed on an MRS agar plate and overlaid with MRS soft agar inoculated with 1% L. delbrueckii subsp. lactis ATCC4797 to assess the bacteriocin crude extracts’ antimicrobial activity.
The bacteriocin crude extract sample was purified with column chromatography using octyl-sepharose (Octyl-sepharose 4 fast flow, Sigma-Aldrich; Merck KGaA). The column was eluted with distilled water and gradient ethanol at a flow rate of 1 mL/min. The purified bacteriocin fraction’s absorbances were measured at 220 and 280 nm. In addition, the bacteriocin activity of each fraction was tested using the spot-on-lawn assay.
The bacteriocin crude extract samples were heated at 65°C and 75°C for 30 min, 85°C and 90°C for 10 and 15 min, 95°C for 20 min, and 100°C for 10, 15, and 30 min to test the bacteriocin’s thermal stability. In addition, the bacteriocin samples were adjusted to pH 3, 4, 5, 6, 7, 8, and 9 to test its pH stability.
A single culture was inoculated into 10% skim milk to identify JNU533’s milk fermentation characteristics. For the pre-culture, 1 mL of JNU533 was incubated in MRS broth (1%, 37°C for 24 h) and then inoculated with 10% skim milk (+0.5% yeast extract, 0.5% glucose) overnight. Then, 5 mL of the pre-culture was inoculated into 10% skim milk (+0.5% glucose for 24 h) medium. Subsequently, 50 mL of the culture medium was sampled every 4 h to determine the pH and colony-forming units (CFU).
The standard is mixture of the following compounds is used: citric acid (HCit) 1 mg/mL, lactic acid (HLac) 25 mg/mL, and glucose 5 mg/mL. Analytical samples are made of 1.5 mL tested-sample+2.55 mL H2 SO4/EDTA mixture (2 mL 0.4 NH2SO4+500 μL H2O+50 μL 5% EDTA). After adding the acid/EDTA mixture to the sample, mix and centrifuge 1 mL in a serum capped vial for the auto sampler. The prepared sample is measured at a UV wavelength of 220 nm. The analysis conditions were as in Table 1.
Column | HPX 87H (Biorad, 40×300 mm) |
Mobile phase | 8 mN H2SO4, 0.01% NaEDTA |
Flow rate | 0.6 mL/min (∼70–80 Bar) |
Temperature | ∼22°C |
Injection Vol. | 10 μL |
Results
The JNU533 strain was isolated from milk products. The genomic DNA of the JNU533 was extracted using the PureHelix™ Genomic DNA Prep Kit and analyzed by 16S rRNA sequencing and BLAST. The isolate was identified as L. lactis subsp. hordniae JNU533. An additional PCR confirmed that the bacteriocin produced by JNU533 was not nisin, as shown in Fig. 1.
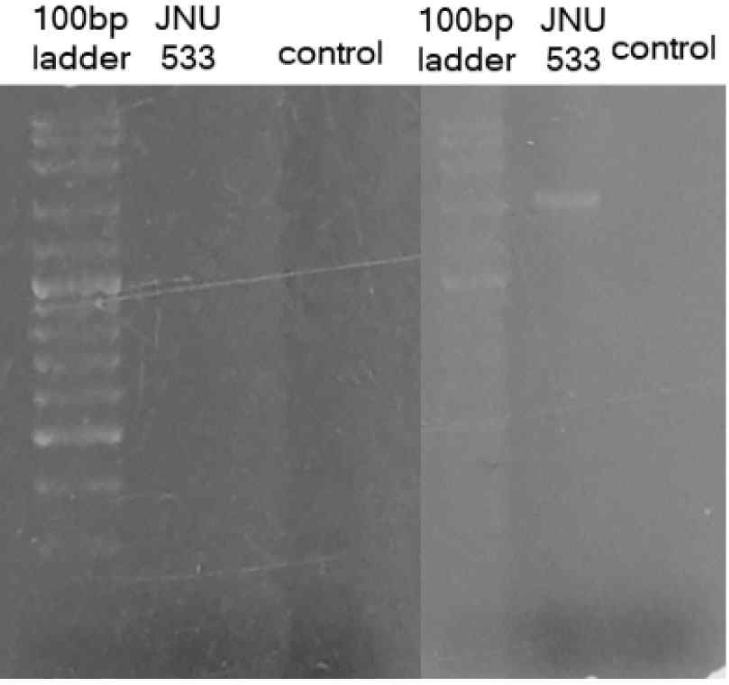
Spot-on-lawn assays were used to determine the bacteriocin activity spectrum. Bacteriocins exhibit inhibitory activity against LAB. The activity units were measured with the highest dilution point that showed a clear inhibitory zone (Table 2).
First, ammonium sulfate precipitation was conducted to obtain the bacteriocin crude extract, and the molecular weight was determined by tricine-SDS-PAGE. The molecular weight of bacteriocin was about 4.9 kDa (Fig. 2). The band on the gel was overlaid on an MRS agar plate of the indicator L. delbrueckii subsp. lactis ATCC4797 to check for the presence of bacteriocin. Fig. 2 shows the bacteriocin activity of the bacteriocin crude extract.
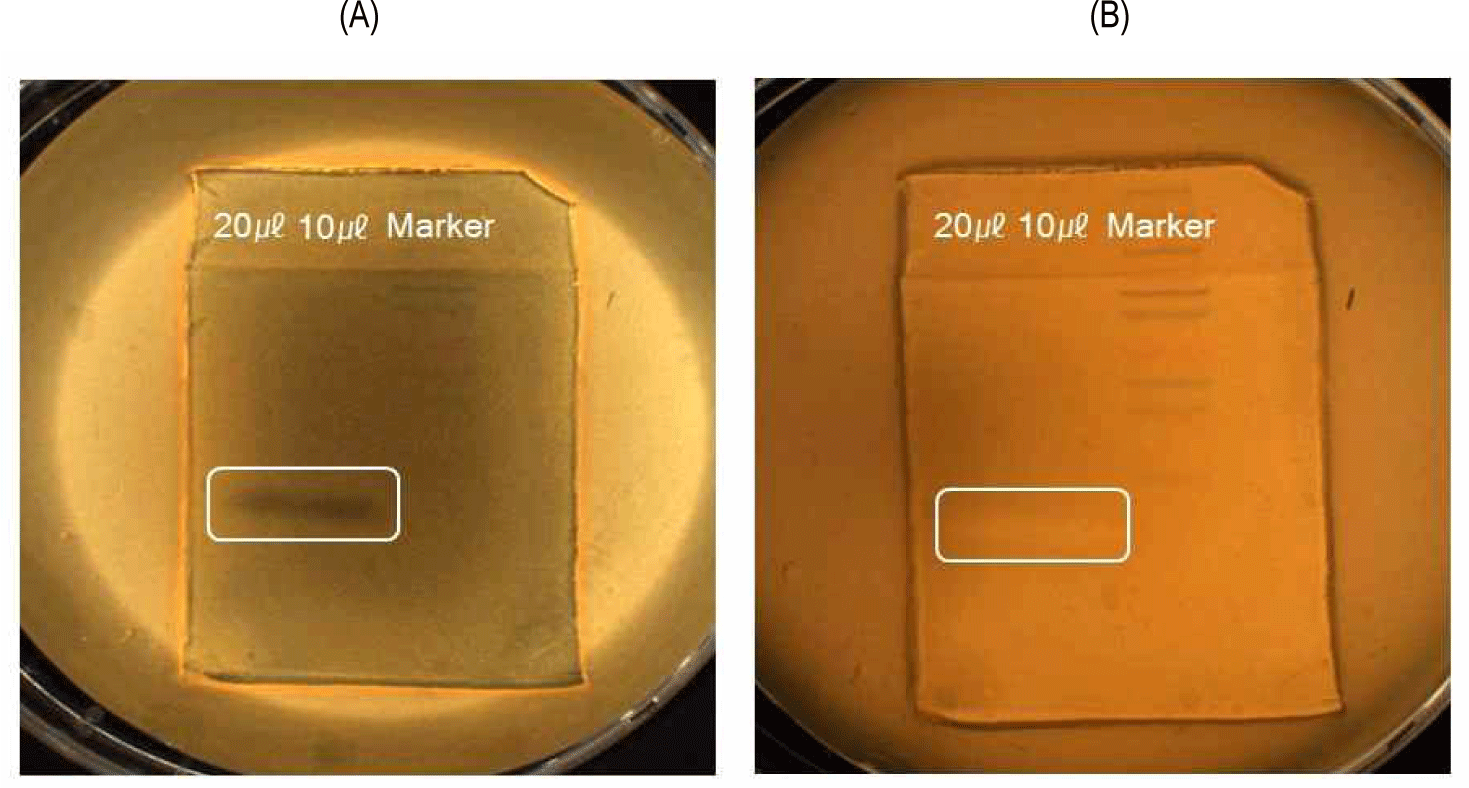
After the bacteriocin crude extract sample was purified by column chromatography, its absorbance was measured at 220 and 280 nm (Fig. 3). Every purified bacteriocin fraction was confirmed using a spot-on-lawn assay.
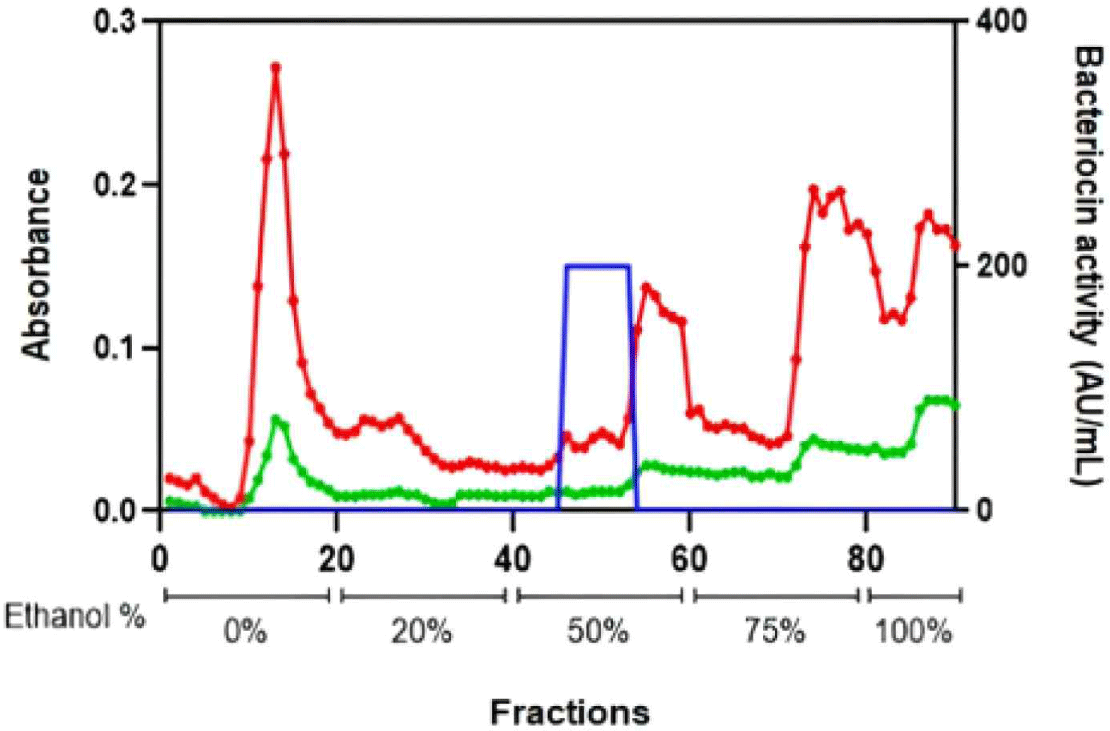
The JNU533 bacteriocin crude extract was heated under various conditions and adjusted to different pH values to determine its heat and pH stability. The results showed that the bacteriocin extract was heat- and pH-stable (Table 3).
A single culture experiment was conducted to determine the characteristics of JNU533 during milk fermentation, using a 10% skim milk and 0.5% glucose medium. The culture medium was sampled every 4 h to measure the pH and viable cell count (CFU/mL; Fig. 4). The results showed that JNU533 grew above 108 CFU/mL. However, the pH decrease was slight (Fig. 4).
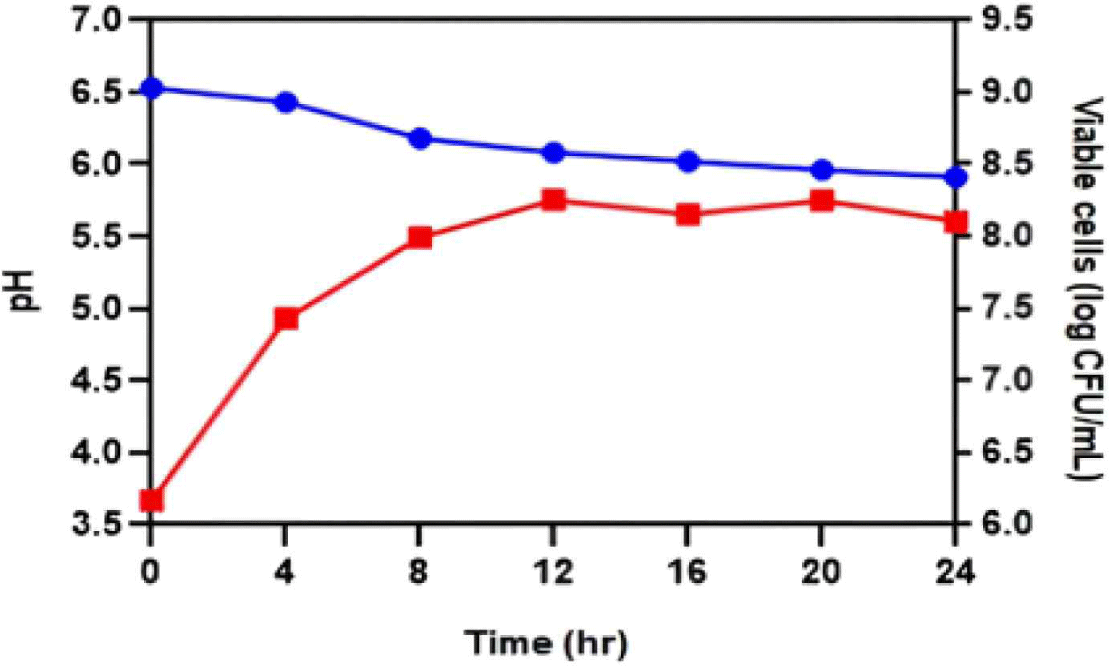
The results showed that, instead of using JNU533 as a starter alone, it should be used with other LAB. Therefore, a mixed culture experiment of JNU533 with L. delbrueckii subsp. bulgaricus was performed. In addition, a single culture fermentation of L. delbrueckii subsp. bulgaricus was conducted (Fig. 5).
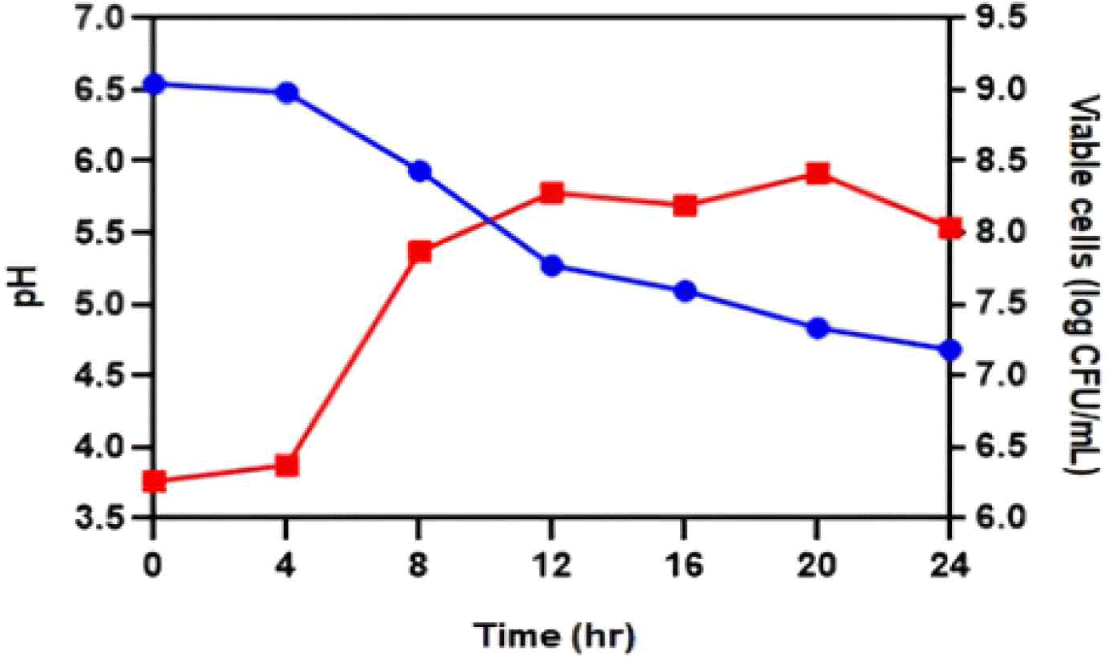
As a result of HPLC analysis, the production of lactic acid increased rapidly from 3 to 9 h fermentation and was maintained at about 0.5% up to 24 h. The production of citric acid was maintained at about 0.1% for 24 h during fermetation. Glucose concentration decreased from 0.67% to 0% over 12 h fermentation (Fig. 6).
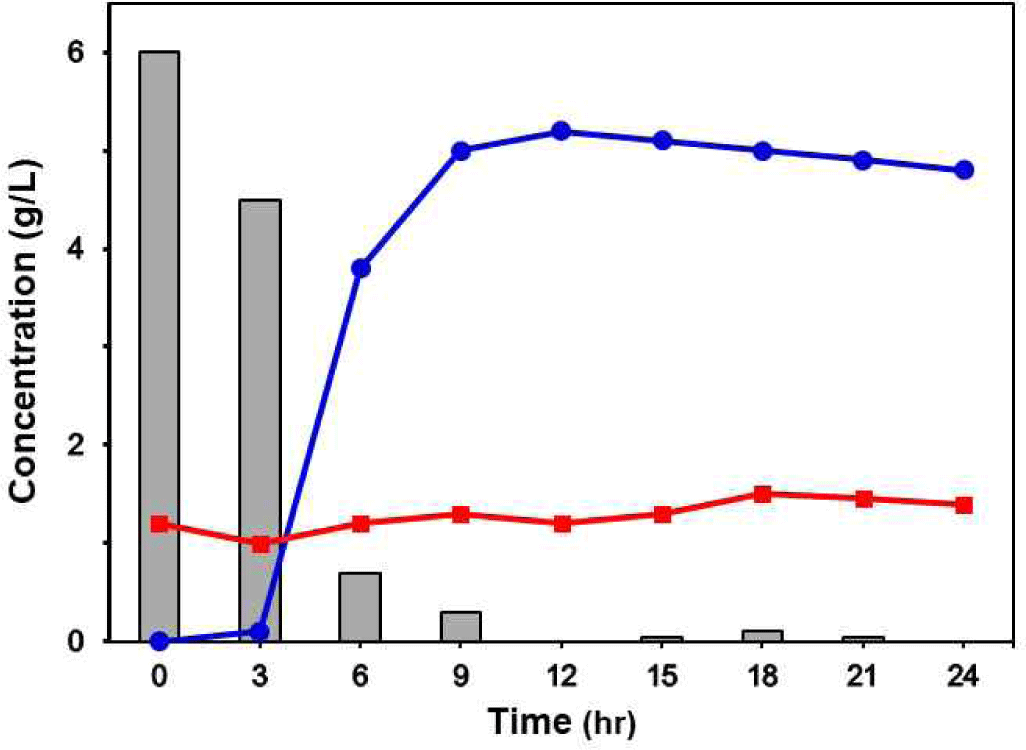
Discussion
Bacterial antibiotic resistance is a global health concern. Therefore, developing new types of antimicrobial agents is increasingly important. Bacteriocins are antibacterial peptides currently being investigated as food additives [12,13], as they are not retained in the human body and are non-toxic, colorless, and odorless. Typically, bacteriocins exhibit stable activity across a diverse pH range with a broad activity spectrum against Gram-positive bacteria [18] and similar strains [11]. Previous studies have found that bacteriocins are not usually active against Gram-negative bacteria.
Lactococcus is a genus of LAB that can produce bacteriocins. In the present study, L. lactis subsp. hordniae JNU533 was isolated from milk products, and its bacteriocin-producing ability was confirmed using L. delbrueckii subsp. lactis ATCC4797. The bacteriocin produced by JNU533 was shown to inhibit LAB, including L. brevis BMB5, BMB6, OB1, OB3, and OB4, L. acidophilus GP1B, L. plantarum L67, OY1, and OY2, L. fermentum, S. thermophilus, and L. delbrueckii subsp. bulgaricus. The JNU533 bacteriocin’s size was found to be 4.9 kDa using tricine-SDS-PAGE, and it was demonstrated to be heat- and pH-stable.
In addition, L. lactis subsp. hordniae, unlike L. lactis subsp. cremoris and L. lactis subsp. lactis, has not been extensively investigated as dairy product starter culture [4]. Therefore, this study aimed to determine the characteristics of JNU533 cultured in milk and the possibility of its application as a starter. A single culture of JNU533 was performed in 10% skim milk medium containing 0.5% glucose (Fig. 4); the JNU533 grew to more than 108 CFU/mL but only decreased the pH slightly.
In conclusion, L. lactis subsp. hordniae JNU533 produced a bacteriocin with antimicrobial activity and showed potential as a starter culture for fermented dairy products.