Introduction
High-protein foods are widely consumed for health purposes, and milk protein is an ideal ingredient owing to its nutritional benefits. Milk protein isolate (MPI) is a representative milk protein powder with a protein level above 90% on a dry matter basis that can provide all essential amino acids and valuable minerals, such as calcium and magnesium [1,2]. MPI contains both slow-acting protein (casein) and fast-acting protein (whey), which can supply sustained amino acids. Owing to these advantages, the demand for MPI is rapidly increasing with the growth of the high-protein food market [2,3]. MPI is generally manufactured by spray drying after removing lactose and mineral salts via ultrafiltration [1,4]. However, spray-dried MPI powder has poor flowability and wettability owing to its fine particle size and dense structure, which can lead to slow rehydration and lump formation [5,6].
Fluidized bed agglomeration can be defined as the size enlargement process from small fine particles to agglomerated large particles that is mainly used to improve the physical properties, such as flowability and wettability, of spray-dried powders with fine particles [7,8]. Currently, high-protein dairy powders are consumed in Korea to promote muscle synthesis and prevent muscle loss, and are generally manufactured by blending spray-dried protein and dairy-based powders with some nutritional ingredients, such as vitamins and minerals [9,10]. Thus, fluidized bed agglomeration is required to improve the physical properties of high-protein dairy powders produced using the dry blending method. Some researchers have studied the effects of the fluidized bed agglomeration on dairy powders such as MPI and skim milk powder (SMP). Ji et al. [5] reported the improved wettability of MPI powders agglomerated by fluidized bed agglomeration, and Turchiuli et al. [11] observed the agglomerate growth of SMP particles during the fluid bed agglomeration process. However, the effect of the fluidized bed agglomeration process in the mixed system of MPI and SMP has not yet been studied. Therefore, the aim of this study was to investigate the effects of fluidized bed agglomeration on mixtures of MPI and SMP at different MPI/SMP ratios.
Materials and Methods
MPI (1900; 85% protein) was supplied by Vitalus Nutrition (Abbotsford, Canada), and SMP containing 37% protein and 49% lactose was obtained from Seoul Dairy Cooperative (Ansan, Korea). The mixtures of MPI and SMP were blended at ratios of 0:10 (SMP), 1:9 (MPI1/SMP9), 2:8 (MPI2/SMP8), and 3:7 (MPI3/SMP7) using a tumbler mixer (Bio- Components Inversina 2 L, Switzerland) for 30 min.
MPI/SMP mixture (1 kg) was placed in a product chamber and agglomeration was performed using a laboratory-scale fluidized bed granulator (Fluid Bed Lap System; Einsystem, Korea). Distilled water (binder solution) was pumped into the spray nozzle at a flow rate of 10 mL/min and sprayed at a pressure of 1.5 bar, and the product temperature was maintained at 55±1°C during agglomeration. After spraying with distilled water for 40 min, the agglomerated powders were dried in hot air at 55°C for 10 min.
The particle size distributions of the fine and agglomerated MPI/SMP mixtures were determined using a laser diffraction particle size analyzer (Mastersizer 3000; Malvern Instruments, UK). The D[3,2] (surface-weighted mean) and span (dispersion index) values were used to interpret the particle size distribution of the agglomerated MPI/SMP mixtures, calculated using Equations (1) and (2), respectively:
where di is the average diameter of the i-th class interval and ni is the number of particles with diameter di. Dv10, Dv50, and Dv90 are the average particle sizes at 10%, 50%, and 90% of the cumulative size distribution, respectively.
Bulk density (ρbulk) and tapped density (ρtapped) of the MPI/SMP mixtures were measured by the method of Wu et al. [6] with slight modification using a tap density meter (BT-301; Bettersize Instrument, China). The ρbulk and ρtapped values were calculated as the mass/volume ratios of the powder samples before and after 1250 taps, respectively.
The flowability and cohesiveness of the MPI/SMP mixtures were evaluated using the Carr index (CI) and the Hausner ratio (HR) [7]. The CI and HR values were calculated using ρbulk and ρtapped using Equations (3) and (4), respectively:
The classification of powder flowability (%) and cohesiveness based on CI and HR values are presented in Table 1.
Classification | ||
---|---|---|
CI (flowability) | <15 | Very good |
15–20 | Good | |
20–35 | Fair | |
35–45 | Bad | |
>45 | Very bad | |
HR (cohesiveness) | <1.2 | Low |
1.2–1.4 | Intermediate | |
>1.4 | High |
The wetting time (tw) was measured to evaluate the rehydration properties of fine and agglomerated MPI/SMP mixtures. According to the method of Atalar and Yazici [7], the wetting time was determined as the time required to complete wetting and immersion of 5 g of powder in 100 mL of distilled water (24±1°C).
Results
The particle size distribution profiles of the fine and agglomerated MPI/SMP mixtures with different MPI ratios are shown in Fig. 1. The fine powder samples exhibited monomodal distributions, which moved to a large region after the fluidized bed agglomeration process. These results indicate that the particle size of the powders increased as they agglomerated during the fluidized bed agglomeration process.
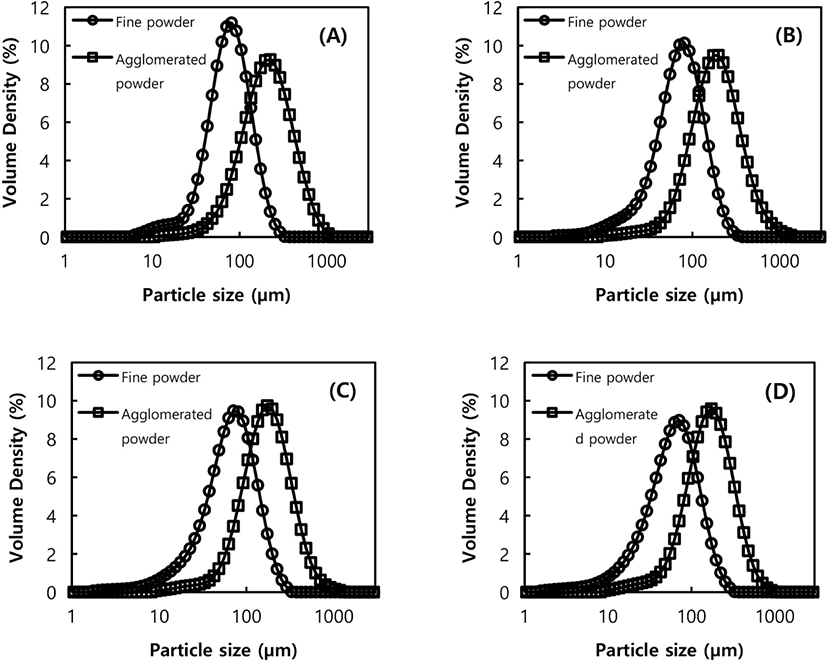
The average particle diameter and distribution of the powder were characterized using the D[3,2] (surface-weighted mean) and span values, respectively. As shown in Table 2, the D[3,2] value of fine SMP was 58.0 μm, which decreased to 35.7 μm (MPI3/SMP7) with an increase in the MPI ratio due to the small particle size of fine MPI (22.7 μm). After the fluidized bed agglomeration process, the D[3,2] values of the MPI/SMP mixtures increased. The span values of the fine MPI/SMP mixtures increased from 1.48 to 1.90 as the MPI ratio increased due to the high span value of fine MPI (2.07), indicating a broad size distribution and low homogeneity. Except for the agglomerated MPI1/SMP9 mixture, the span values of the agglomerated MPI/SMP mixtures were lower than those of the non-agglomerated MPI/SMP mixtures, indicating a narrow size distribution with homogenous powders.
Sample | D[3,2] (μm) | Span | |
---|---|---|---|
Fine powder | MPI | 22.7±0.40a | 2.07±0.02a |
SMP | 58.0±0.85b | 1.48±0.01b | |
MPI1/SMP9 | 47.8±1.26c | 1.67±0.01c | |
MPI2/SMP8 | 40.4±0.15d | 1.79±0.01df | |
MPI3/SMP7 | 35.7±0.15d | 1.90±0.01e | |
Agglomerated powder | SMP | 151±3.79e | 1.81±0.03df |
MPI1/SMP9 | 136±1.00f | 1.82±0.04f | |
MPI2/SMP8 | 127±1.73g | 1.75±0.02df | |
MPI3/SMP7 | 118±1.15h | 1.75±0.03d |
The densities of the fine and agglomerated MPI/SMP mixtures with different MPI ratios are shown in Fig. 2. The bulk density (ρbulk) and tapped density (ρtapped) values of the fine powder samples were in the range 0.40–0.47 and 0.54–0.58 g/mL, respectively. After the fluidized bed agglomeration process, ρbulk and ρtapped values decreased to 0.26–0.32 and 0.31–0.38 g/mL, respectively, and decreased with an increase in the MPI ratio.
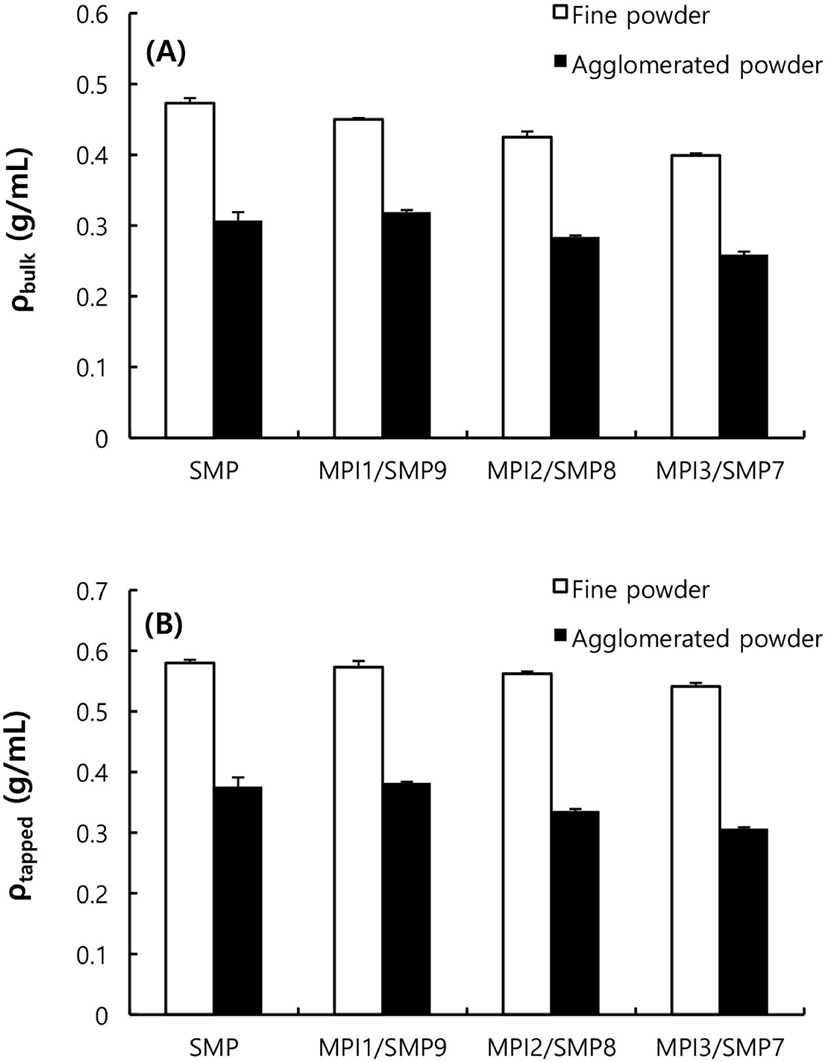
The flowability and cohesiveness of food powders can be characterized using CI and HR values, respectively. As shown in Table 3, the CI value of the fine SMP sample showed good flowability (CI: 15–20) and increased from 18.4 to 26.3 as the MPI ratio increased, indicating fair flowability (CI: 20–35). The HR value of the fine SMP sample was 1.23 and that of the fine MPI/SMP mixtures increased with increasing MPI ratio, indicating intermediate cohesiveness (HR: 1.2–1.4). In contrast, the CI and HR values of the agglomerated MPI/SMP mixtures showed good flowability (CI<15) and low cohesiveness (HR<1.2). These results indicate that the physical properties of MPI/SMP mixtures can be effectively improved via fluidized bed agglomeration.
The wettabilities of the fine and agglomerated MPI/SMP mixtures with different MPI ratios are listed in Table 3. The fine powder samples exhibited a long wetting time (tw) >120 s and floated on the water surface with lump formation. In contrast, the wetting time of agglomerated powder samples significantly decreased to 4.67–58.3 s. With the exception of the MPI3/SMP7 sample, agglomerated powder samples exhibited a faster wetting time than the instant criterion (<20 s) described by Chever et al. [12].
Discussion
Particle size distribution is an important property that is closely related to other physical properties, such as density, flowability, and wettability, of powdered products. As evidenced by the increased particle size, the fine powder samples were effectively agglomerated by the fluidized bed process. This agglomeration of fine powders can be attributed to the formation of solid bridges between fine particles during the fluidized bed process. In general, the fluidized bed agglomeration process is divided into three steps: wetting, coalescence, and consolidation. The fine powders were fluidized by hot air and their surfaces were wet by the sprayed binder solution. The wet powder particles collided with each other and bound together, leading to the formation of liquid bridges. Subsequently, the liquid bridges become solid bridges by hot air, which leads to the consolidation of agglomerated particles [13,14]. Therefore, the fluidized bed process can effectively increase the particle size and produce agglomerated powders.
The main purpose of the fluidized bed agglomeration process is to achieve uniform particle size and distribution. Span value is generally used to evaluate the homogeneity and polydispersity of food powders. The high span value of the agglomerated SMP sample may be attributed to the high friability of the agglomerated SMP particles owing to friction within the fluidized bed [15,16]. In contrast, the span value of the agglomerated MPI3/SMP7 sample was significantly lower than that of the non-agglomerated MPI3/SMP7 sample. This reduction in the span value indicates that the broad distribution of the fine powders was transformed into a narrow size distribution with homogenous powders via fluidized bed agglomeration. From these results, it can be concluded that the particle size distribution of the MPI/SMP mixtures can be effectively improved using the fluidized bed agglomeration process.
Fluidized bed agglomeration led to a lower density than that of the non-agglomerated powder samples. This result can be attributed to the increased particle size and porous structure of the agglomerated powders, which can cause additional voids and result in a lower density [5,12]. In contrast, the fine powder samples showed higher densities owing to their dense structures. Consequently, the decreased ρbulk and ρtapped values of the agglomerated MPI/SMP mixtures can be explained by the additional voids within the porous structure of the agglomerated powders.
CI and HR values of the MPI/SMP mixtures were significantly improved via fluidized bed agglomeration. The improved flowability and cohesiveness of the agglomerated powders can be explained by the increased particle size, which can decrease the friction between the particles owing to the reduction in the particle surface area [14,17]. Fluidized bed agglomeration also improved the wettability of the MPI/SMP mixtures. This improved wettability can be attributed to the porous structure of the agglomerated powder. The large porous structure allows water to easily penetrate into the void spaces of the agglomerated particles, facilitating the wetting of the powders [6,8]. These results suggest that the physical properties, such as flowability and wettability, of the MPI/SMP mixtures can be effectively improved to obtain increased particle size and porous structure via the fluidized bed agglomeration process.
Conclusion
The high-protein dairy powder is being consumed to promote muscle synthesis and prevent muscle loss. However, spray-dried milk proteins have poor flowability and wettability owing to their fine particle sizes and high inter-particle cohesive forces. To resolve these problems, this study investigated the effect of fluidized bed agglomeration on MPI/SMP mixtures with varying MPI/SMP ratios. The fluidized bed process increased the particle size from 35.7–58 μm to 118–136 μm, and both CI and HR values of MPI/SMP mixtures decreased from 21.4–26.3 and 1.27–1.36 to 15.7–16.3 and 1.18–1.19, respectively. These results showed that the flowability level was improved from fair (20–35) to good (15–20) and cohesiveness level was also changed from intermediate (1.2–1.4) to low (<1.2) after the fluidized bed agglomeration. Moreover, the wetting time of agglomerated MPI/SMP mixtures was effectively reduced to 4.67–58.3 s by the fluidized bed agglomeration. In particular, agglomerated MPI1/SMP9 and MPI2/SMP8 samples exhibited a faster wetting time than the instant criterion (<20 s). The results of this study may be useful in manufacturing protein-enriched dairy powders with good instant properties.